Fundamental physics on ion-photon interaction is studied to realize the ion-photon interface, which is a basic building block of the ion-photon quantum network. The technologies established in the course to this goal are deployed to the research towards the ultimate ion-trap frequency standards based on quantum logic spectroscopy.
Principal investigator; K. Hayasaka
Home > About NICT > Organization > Advanced ICT Research Institute > Quantum ICT Advanced Development Center > Ion-photon quantum network
The system consisting of trapped ions as pictured in figure.1 is one of the most promising candidates of large-scale quantum computation. So far small-scale quantum computation with up to eight ions has been realized. Networking of the small-scale trapped-ion quantum computers is of crucial importance in establishing fault-tolerant large-scale quantum computation. A basic element to this end is the quantum interface, in which a ion and a photon interchange quantum states with high fidelity.
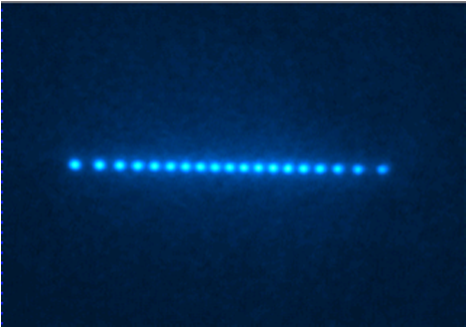
The basic requirement of the ion-photon quantum interface is strong coupling of an ion and an optical cavity. This condition is fulfilled when the exchange of a quantum between an ion and an optical cavity mode evolves faster than the decay of induced dipole in the ion as well as of the cavity-field decay. The strong coupling of an ion and a cavity has not been realized yet, although the research toward this goal has been started in 1990s. The main obstacle is difficulty in reducing the size of the trap electrodes until they are inserted in an optical cavity with a length smaller than 1mm. This length is the upper limit of the strong coupling condition for most of ion species.
An alternative approach to detour this difficulty is to use collective coupling of multiple ions. A system of N ions localized at antinodes of the cavity mode exhibits enhancement of the coupling with a factor of N1/2. A hundred of Ca+ ions might be brought into the strong coupling regime even with a 5-mm cavity. Our effort is focused on achieving this situation. A thin ion trap inserted in between the cavity mirrors shown in fig.2 is an example of our implementation. A systematic approach to the single-ion coupling might be possible by reducing the number of ions as well as the cavity length, starting from this setup.
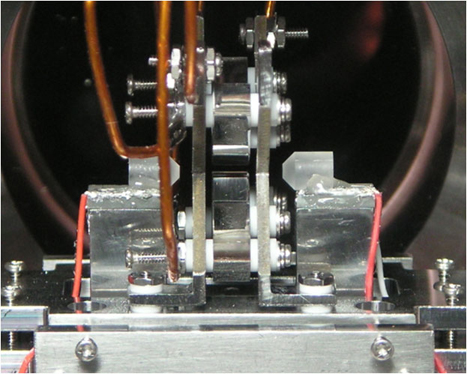
The main concern of research with trapped ions has been the large-scale quantum computation. However, the quantum logic spectroscopy (QLS) developed by NIST group in 2005 has demonstrated the power of small-scale quantum computation. They made a new record of the measurement precision in history by measuring the frequency of Al+ with an uncertainty of 2.3x10-17 using QLS, together with frequency Hg+ with an accuracy of 1.9x10-17. QLS is a kind of small-scale quantum computation with trapped ions. QLS could be applicable to various kind of ions, including molecular ions, with which spectroscopy has been so far impossible. Of particular importance is the alkaline-earth-like ions. Application of QLS to these ions might realize ion frequency standards with an uncertainty of 10-18 level proposed by Nobel Prize laureate H. Dehmelt in 1982. We study QLS on In+ in collaboration with Space-time standards group and Osaka university.
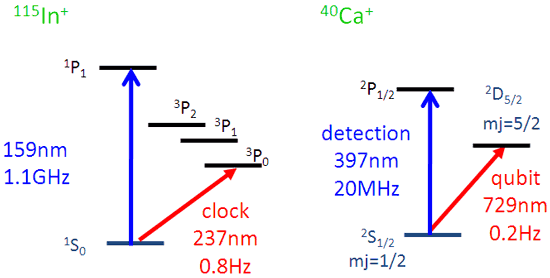
Energy levels of In+ are shown in Fig.3. The clock transition 1S0-3P0(237nm) is immune to various kinds of shifts, and an uncertainty as small as 10-18 is expected. Observation of the transition requires excitation of the 1S0-1P1 by illuminating the ion with coherent radiation at 159nm. However, generation of the light is not possible by existing technologies. The observation could be substituted by that of Ca+ at 397nm, when QLS is applied to the system consisting of In+ and Ca+. The detailed procedure of the observation is described in fig.4 using the notation of quantum circuit diagram.
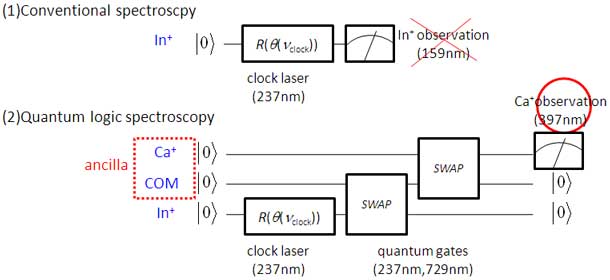
The second is defined by the hyperfine transition of Cs. A new definition is going to being determined using some optical transition in 2019. Various ion and neutral atom species are being studied to be a candidate of the new second. In+ is one of the most promising species as the optical frequency standards, if QLS would be successfully applied to this ion.