In quantum communication, it is important to make special ties called quantum entanglement between multiple, spatially separated signals. The entanglement would be applied to realize massively parallel computing, communications unable to be eavesdropped, and even low-power high-capacity communication. However, because the entanglement is fragile to transmission losses, restoring and even amplifying the strength of the ties (“distillation”) is essential. In particular, in order to implement quantum communications in the current infrastructure of optical communications, the entanglement between more wave-like states must be distilled. This is called “continuous variable entanglement distillation,” which is more difficult than that for the single-photon entanglement, and had not been achieved so far.
Home > Press Release > World’s First Demonstration of Continuous Variable Entanglement Distillation for Quantum Communication
World’s First Demonstration of Continuous Variable Entanglement Distillation for Quantum Communication
- Published in “Nature Photonics” -
National Institute of Information and Communications Technology (NICT, President: Hideo Miyahara) has demonstrated an important milestone protocol, called “entanglement distillation” applicable to continuous variable quantum communications. Quantum entanglement is a strong correlation formed between two or more quantum systems. This can be used to realize secure and high capacity communication that are impossible by classical systems. Because the entanglement is very susceptible to damping, distilling stronger entanglement from the degraded one is indispensable after transmission. While distillation for entangled single photons was previously demonstrated, the distillation for more wave-like, continuous variable (CV) states had not been realized until now. This is important because current optical networks are based on CV technology, and eventually it would deliver ultra-high capacity communications with minimum power in the current infrastructure. This result will be published in the UK science journal, "Nature Photonics."
NICT has successfully performed the world’s first complete distillation of continuous variable entanglement between two wave-like states. NICT's unique technology which can realize subtraction of photons in the signal beams plays a key role here. The conventional optical signal amplification technologies like EDFA cannot amplify a damped quantum entanglement because of inevitable noises. Our technology can in principle recover quantum entanglement in a noiseless manner. If this technology is applied to processing at network nodes, it would be possible to achieve the maximum transmission capacity using a given transmission power.
Increasing the number of entangled signals would enable one to implement quantum computation at the network nodes in the future. In this way, one could achieve maximum capacity communications with the minimum power as indicated by recent theoretical studies.
Hiroki Takahashi, Jonas S. Neergaard-Nielsen, Makoto Takeuchi, Masahiro Takeoka,
Kazuhiro Hayasaka, Akira Furusawa, and Masahide Sasaki, DOI:10.1038/NPHOTON.2010.1.
*We thankfully acknowledge the assistance from the University of Tokyo.
Photons
According to quantum mechanics, light has the properties of waves and particles at the same time. Light particles, called photons, have the smallest unit of light energy which cannot be split further. For example, the photon energy (in Joule) of 1.5 micron wavelength light (typically used in optical communications) is approximately equal to 1 divided by 10 to the 19th power and thus an extremely small value.
EDFA
Erbium Doped Fiber Amplifier is a fiber device for optical signal amplification doped with erbium ions. It can amplify 1.5 micron light and is therefore essential for long-haul optical communication. However, contamination by amplification noise is inevitable, even in principle, and this is one of the factors which limit large-capacity long-distance communication.
Quantum Computation
Whereas the bit values 0 or 1 cannot be used physically at the same time in conventional computation methods, in quantum computation, one can use the superposition state of “0” and “1” at the same time as one bit. Such a bit state is called a quantum bit (qubit), and because of that property it can be utilized for parallel computation. In theory, a quantum computer can solve some complicated problems in as little as several tens of seconds; problems which will take the currently fastest supercomputers thousands of years to solve. At present, many systems using laser-cooled ions, superconducting devices, semiconductor devices, optical quantum circuits, etc. are being developed for realizing quantum computation. Among these, optical quantum circuits are indispensable when used in combination with current optical networks.
Appendix
The restoration and amplification of the strength of quantum entanglement after transmission has previously been demonstrated using quantum entanglement between single photon pulses(1). It was shown theoretically (2) that distillation of quantum entanglement between wave-like signals (continuous-variable quantum entanglement) is impossible to implement with the conventional technology. However, for certain limited kinds of signal degradation, distillation of the entanglement is possible with existing technologies, as was experimentally demonstrated in 2008 (3).
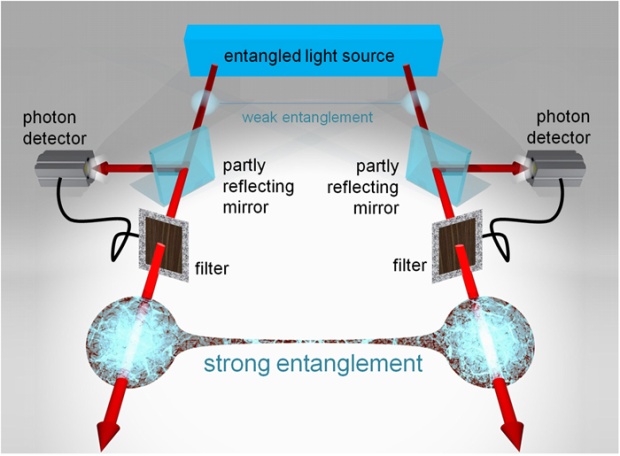
NICT has developed a new technology to allow for the distillation of quantum entanglement for continuous variable signals of any shape (4). At the core of this technology is the step of letting each of the separated entangled light beams pass through partially reflecting mirrors. The small reflections from each mirror are guided towards photon detectors, and only when photons are observed there, the “filters” are opened to let the main signal pass. Through this “filtering” operation, noise and degraded signals are discarded while the entanglement of the remaining signals is increased. Allowing for a certain probability of failure and thereby sacrificing signal rates, this scheme is able to distill the quantum entanglement beyond the capabilities of conventional technologies. Figure 1 shows a conceptual drawing of the distillation process.
The strength of the quantum entanglement shared between the two light beams can be quantified by the so-called logarithmic negativity. Measurement results are shown in Figure 2. The blue points indicate the measurements after distillation, and when comparing with the undistilled measurements (green points), it is clear that entanglement increase has been observed. The “initial squeezing level” on the horizontal axis corresponds to the degree of entanglement of the input signals, so the distillation has been carried out a several different entanglement levels.
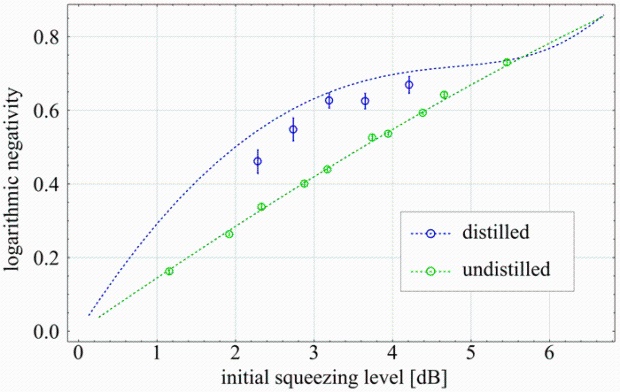
As shown by the blue points, the distillation effect was clearly observed, when compared with the green points before distillation. The values on the horizontal axis are related to the degree of quantum entanglement of the input. The dotted lines are the theoretical curves.
For Technical Inquiries
Hiroshi HARADA, Homare MURAKAMI,
Kentaro ISHIZU
Masahide SASAKI
Quantum ICT Group
New Generation Network Research Center
Tel :+81-42-327-6524
E-mail:
Public Relations
Sachiko HIROTA
Public Relations Office
Strategic Planning Department
Tel:+81-42-327-6923
E-mail: