The performance of optical communications is determined by a bit error rate (BER) in discriminating the signals “0” and “1”. It can be lowered by eliminating noises in the system. It is bounded, however, by an unavoidable noise, called quantum noise. In optical communication theory, it is believed that the BER cannot be lowered below a certain limit (the shot noise limit, SNL). In contrast, quantum communication theory tells us that a BER can be reduced below the SNL by controlling quantum noise. Controlling quantum noise in a receiver, however, has been a formidable task. Hence, none could have beaten the SNL so far.
Home > Press Release > Bit error rate bound of optical communication theory was beaten
- An important step toward ultra-long-distance, low-power and high-capacity communications -
National Institute of Information and Communications Technology (hereinafter called NICT, President: Hideo Miyahara), in collaboration with National Institute of Advanced Industrial Science and Technology (hereinafter called AIST) and Nihon University, has succeeded in the world’s first demonstration of beating the bit error rate bound of optical communication theory, by using a new technology of “quantum receiver”. By replacing a conventional optical receiver with this quantum receiver, one will be able to realize large capacity communications without increasing the signal power in a fiber, as well as to extend a communication distance in space. Our result is an important step toward realizing those tasks. This achievement has been reported over the official website and a magazine of Physical Review Letters*, on June 24, 2011.
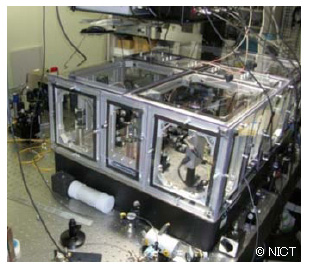
NICT has invented a new quantum receiver to suppress the effect of quantum noise, by introducing a technology to control the particle nature of light (photons), to conventional optical communication technology which controls the wave nature of light. By embedding the photon number detector with the world’s highest-sensitivity superconducting transition-edge sensor developed by AIST and Nihon University in this quantum receiver, the research team was able to beat the SNL of optical communication theory for the first time in the world.
This is a breakthrough toward research and development for ultra-long-distance, low-power and high-capacity communications beyond the limits of optical communication theory.
The quantum receiver will be able to be installed into existing infrastructures of optical networks to realize large capacity communications with much lower power. The first milestone would be an application to enhancing a satellite-ground optical link, in 10 years, which would eventually reduce the number of repeaters in long-distance optical fiber communications, as well as increase the capacity without spending the transmission power in a fiber. Moreover, its ability to detect photons with high precision would be useful for the optical metrology.
* | Information on paper publication |
URL: http://prl.aps.org/ | |
K. Tsujino, D. Fukuda, G. Fujii, S. Inoue, M. Fujiwara, M. Takeoka, and M. Sasaki, "Quantum receiver beyond the standard quantum limit of coherent optical communication," Physical Review Letters 106, 250503 (2011). |
<Outline and experimental results of quantum receiver developed this time>
[ Quantum receiver developed by NICT ]
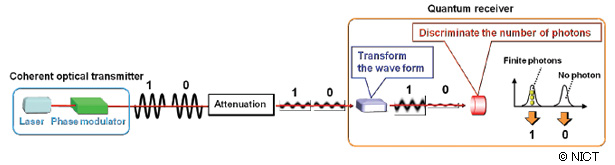
In this experiment, the bit signals “0” and “1” are conveyed by two waves whose shapes are upside down to each other (binary phase-shift keyed (BPSK) signals), and detected by the quantum receiver. (The BPSK signals are the most basic one used in current coherent optical communications.) Instead of directly measuring the waves of “0” and “1”, one first transforms them to another form of light wave, and then measures the number of photons, as shown in Fig. 1. When photons are detected, a decision is made that the signal is “1”, while when no photon is registered, the signal is decided as “0”. This enables the more precise discrimination of the signals used in optical communications, by suppressing the effect of quantum noise. The behavior of photons is in some sense unpredictable as described by the Heisenberg uncertainty principle. The number of photons in the signal pulses generated from laser is fluctuating around a certain average value. As far as one deals with optical signals as waves, one cannot tame this unpredictable behavior of photons. One can minimize, however, the fluctuation of photons by measuring the particle nature of light precisely. This is the key to beat the SNL.
[ Conventional coherent optical receiver ]
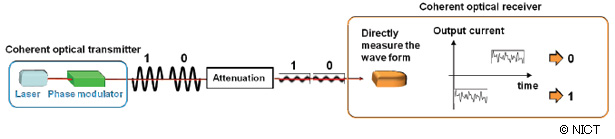
In contrast to quantum receiver, the coherent optical receiver directly measures the wave forms of “0” and “1”. More precisely, the signal is first combined with a reference pulse called the local oscillator, and then converted into an electric current. The signals “0” and “1” are detected as positive and negative currents respectively. At this detection process, even an ideal coherent optical receiver suffers from the bit error rate bound (the SNL) imposed by the quantum noise.
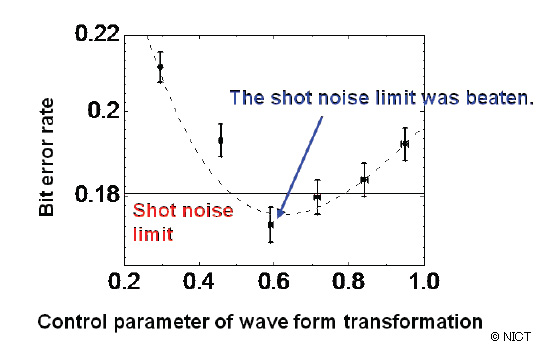
The result of discrimination experiment of faint signals whose average photon number is 0.21 for both “0” and “1” is shown in Fig. 3. The vertical axis is the bit error rate, while the horizontal axis is the control parameter of wave form transformation (the amount of the shift in the average photon number of the bit signal “0”). The bit error rates of such weak signals are high, and the bound in optical communication theory (the SNL) is 0.18 (the red horizontal line). The bit error rate attained by the quantum receiver was minimized to be 0.174 for the control parameter of 0.6, demonstrating the lower value below the SNL.
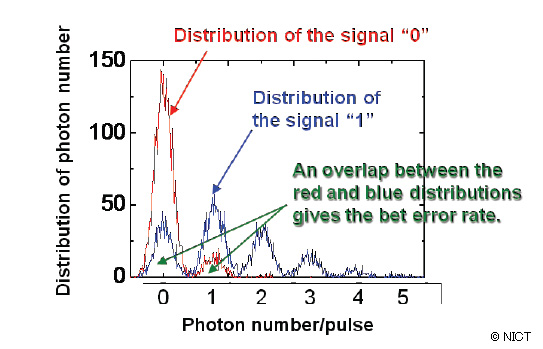
In Fig. 4, distributions of output photon numbers for the signals “0” and “1” are shown. The signal “0” is detected as a state close to the vacuum (red), while the signal “1” is detected a state which includes multiple photons (blue). The bit error rate is given by an overlap between these two distributions.
The visibility of the signal and the local oscillator light was as high as 98.6%, and the total photon detection efficiency was as high as 91%, thanks to the state-of-the-art technology of coherent light control developed by NICT, and to the photon number detector developed by AIST and Nihon University. Quantum receiver built with these technologies enabled clear discrimination of photon numbers as seen in Fig. 4, and led to surpassing the bit error rate bound of optical communication theory.
Technical Contact
Quantum ICT Laboratory,
Advanced ICT Research Institute
Tel:+81-42-327- 6524
E-mail:
Media Contact
Public Relations Department
Tel:+81-42-327-6923
E-mail: