Chip-Level-Integrated Frequency Standards
While almost all accurate frequency standards remain laboratory-sized devices, it is continued miniaturization and cost reduction drives the revolutionary technological progress that is most likely to affect our every-day lives.
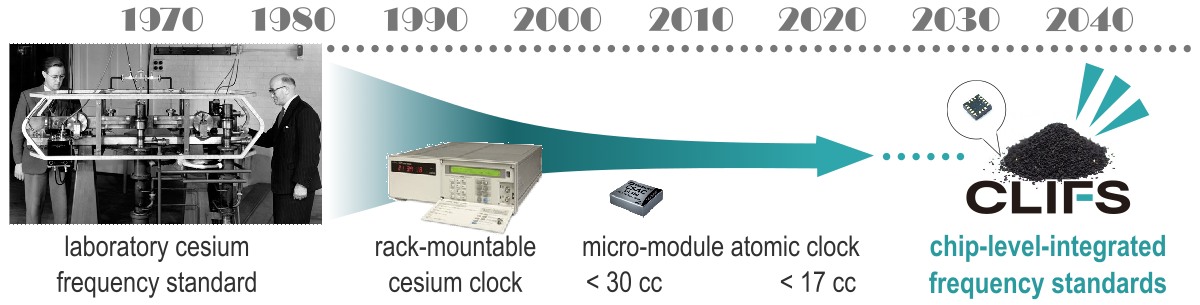
Microwave atomic clocks have defined the SI second since 1967. Today, a handful of metrological institutes world-wide operate approximately a dozen hand-built, highly accurate primary frequency standards to ensure that international time follows this definition. But even these institutes rely on smaller, more robust commercial cesium clocks to generate the time signals that they make available to society. Multiple of these clocks can fit on a shelf, and they are much more easily replaced in case of failure. Further development into compact, low-power modules made it possible to integrate atomic clocks in the satellites of the Global Positioning System (GPS) that created a new era of navigation. With reduction in cost, atomic clock modules are now integrated in the base stations of telecommunications systems, providing the precise timing needed for high bandwidth networks. But even today, atomic clocks are too bulky, expensive and power-hungry to include them in consumer devices like mobile phones.
We are creating technology to overcome this final “cliff” in the development of atomic frequency standards and bring them to a size and price-point comparable to modern microprocessors.
As communication networks evolve from mere telephony to including diverse applications like automobiles, robots or aerial drones, precise timing sources at the endpoint will help provide the required efficiency, security and stability for future applications. Future applications will depend on such devices to maintain authenticated time and position even when the signals of global navigational satellite systems are unavailable.
Clock components
Creating such Chip-Level-Integrated Frequency Standards (CLIFS) requires miniaturizing multiple integral components of an atomic clock in a way that is suitable for mass production. An atomic clock usually requires
- A method to interact with the selected kind of atom and probe its response
- A sample of atoms that react to a resonant excitation at a precise frequency
- A suitable frequency source that can be adjusted to match the atomic resonance
Laser source
A vertical-cavity surface-emitting laser (VCSEL) is a convenient, power-efficient source of excitation. Thousands of such devices can be produced simultaneously in a semiconductor process based on gallium arsenide. The atomic response to the emitted laser light can be directly measured with a photo detector.
The most accurate atomic clocks now directly probe optical atomic resonances, but the laser stability required for this is not yet available outside of laboratory applications. The type of clock we are exploring instead applies an additional modulation to the laser, and it is this frequency of several GHz that probes the selected atomic resonance in a process known as coherent population trapping (CPT).
However, the laser still needs to be kept near a wavelength where it interacts with the atoms. This is often achieved by using a controlled source of heat to cause the structures defining the wavelength of the laser to expand as needed, but this requires a constant supply of energy just to maintain the correct working point. Instead, we are preparing a laser that can be reliably manufactured with a precise length of the laser cavity. It then uses micro-electromechanical systems (MEMS) to fine-adjust this length and the resulting wavelength of the laser emission. By using electrostatic forces acting on a membrane that holds the cavity mirror, no energy needs to be supplied to maintain the current wavelength, only to actively change it.
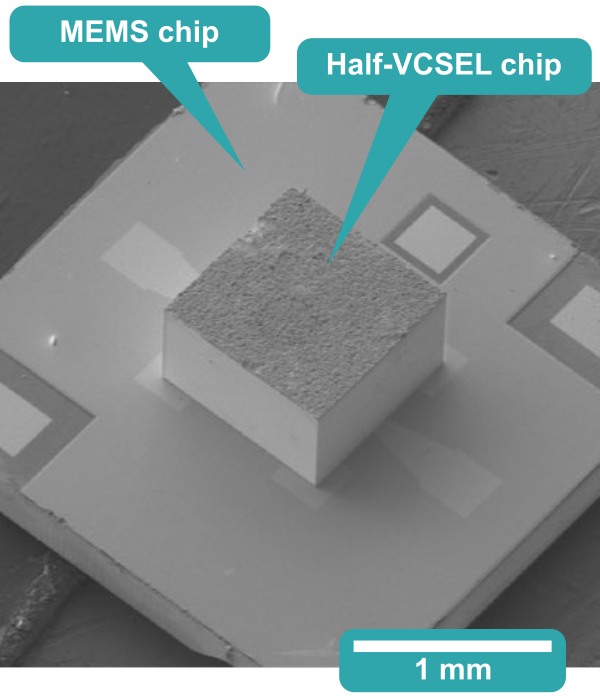
Gas cell
The atomic sample is prepared in a miniaturized gas cell built up in several layers of silicon and glass components. To provide a compact package, the laser and photodetector are mounted on the same side of the cell, with two mirrors deflecting the light to travel along the long axis of the cell and interact with a large number of atoms. A source of rubidium is permanently enclosed in the cell, and a buffer gas composed of nitrogen and argon minimizes the interaction of the rubidium atoms with the cell walls. The entire structure is only a few millimeters tall.
FBAR oscillator
The signal source we are developing for a CLIFS device is a thin-film bulk acoustic resonator (FBAR). This is a mechanically resonating structure consisting of a piezoelectric material covered by metallic electrodes on both sides. Unlike a quartz oscillator, the FBAR can be fabricated entirely in a CMOS (complementary metal-oxide semiconductor) process, which allows the production of precise structures only a few micrometers in size. These structures can reach the resonant frequencies of 3.4 GHz or 4.6 GHz required for the laser modulation in rubidium- or cesium-based clocks. The CMOS process can also produce the integrated circuitry needed to power and fine-tune the oscillation of the resonator.
In our experiments a CMOS circuit controls the frequency of the FBAR oscillator and amplifies the signal so that it can be used to modulate the laser. In a future CLIFS device, these can be manufactured together on the same substrate and integrated into a single package only a few mm in size.
Outlook
We believe that once Chip Level Integrated Frequency Standards, as robust, drift-free atomic clock devices become available at a size and cost comparable to modern micro-processors, they will find wide use in timing-critical applications that now rely on GPS signals. They will enable communications protocols for Beyond 5G and 6G networks and may create new applications that are not possible or cost effective with current technology.
References
Overview (Japanese)
1)
M. Hara,
Perspective スマホに載る原子時計 実現へのシナリオ,
日経BP日経エレクトロニクス 1219, 85
(2020)
2)
M. Hara,
Perspective 原子時計のチップ化が導く 超高精度デジタルツイン,
日経BP日経エレクトロニクス 1217, 81
(2020)
Laser source
3)
Z. Zhao, M. Toda, T. Ono, M. Hara, S. Shinada, H. Nakagawa and K. Kikuchi,
800 nm band MEMS-tunable VCSEL for microfabricated atomic clock,
in Proc. Smart System Integration (SSI), 26-28 April 2022, Grenoble, to be published in
IEEE Xplore
(2022)
Gas cell
4)
H. Nishino, Y. Yano, M. Hara, M. Toda, M. Kajita, T. Ido and T. Ono,
Reflection-type vapor cell for micro atomic clocks using local anodic bonding of 45° mirrors,
Optics Letters 46(1), 2272-2275
(2021)
5)
H. Nishino, M. Hara, Y. Yano, M. Toda, Y. Kanamori, M. Kajita, T. Ido and T. Ono,
A reflection-type vapor cell using anisotropic etching of silicon for micro atomic clocks,
Applied Physics Express 12(7), 072012
(2019)
FBAR oscillator
6)
M. Hara, Y. Yano, Y. Takahashi, T. Nishizawa, S. Hara, A. Kasamatsu, M. Ueda, H. Ito and T. Ido,
Super-high-frequency-band injection-locked two-divider oscillator using thin-film bulk acoustic resonator,
Electronics Letters 57(3), 132-134
(2021)
M. Hara, Y. Yano, M. Kajita, H. Nishino, Y. Ibata, M. Toda, S. Hara, A. Kasamatsu, H. Ito, T. Ono and T. Ido,
Microwave oscillator using piezoelectric thin-film resonator aiming for ultraminiaturization of atomic clock,
Review of Scientific Instruments 89(10), 105002
(2018)